To view a pdf version of this Expertise Note, view the Library resource here.
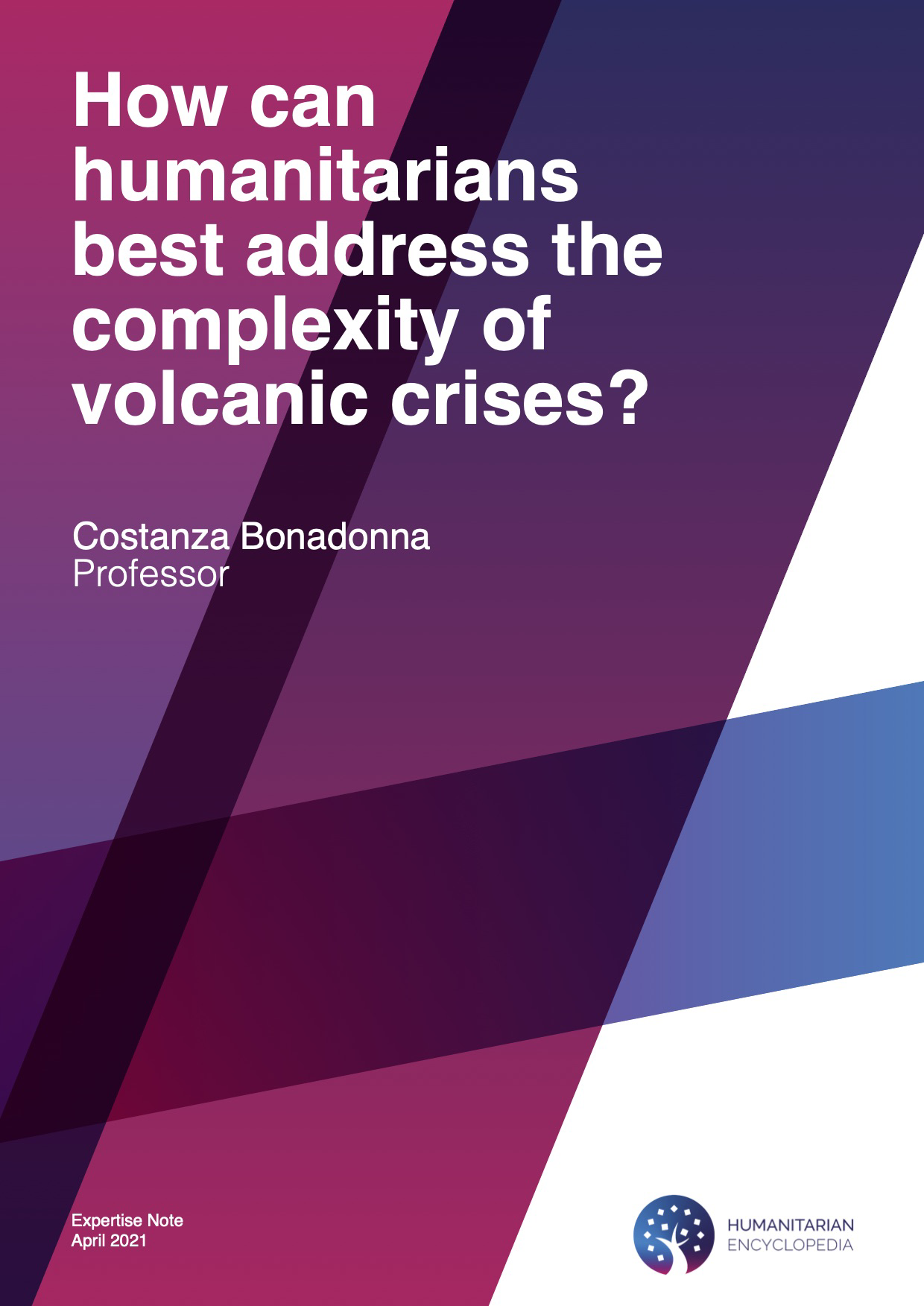
Disaster Risk Reduction (DRR) and other related concepts such as prevention and response cannot be achieved without appreciating the complexity of natural phenomena. Even within a phenomenon as specific as volcanic eruptions, there is a great deal of variation in the dynamics, hazards, damage agents, impacts and responses needed. In this article I summarise key scientific aspects and present a series of case studies to best illustrate this complexity.
Volcanic hazards and their impacts
Spatial and temporal distribution of volcanic hazards
Worldwide nearly 60 million people live within about 10 km of a volcano that has erupted in the last 10,000 years, but more than 1 billion live within 100 km (Freire et al., 2019). Several volcanic hazards are capable of reaching these distances (e.g. lahars, volcanic gases) and beyond (ash dispersal and fallout). As a result, 14% of the global population is at risk from the effects of a volcanic eruption and the numbers are likely to continue to increase above population growth rates, exacerbating the management of humanitarian crises in some instances.
One important aspect when considering humanitarian aid for these populations is that unlike some other natural hazard events (e.g., earthquakes, landslides/rockfall, avalanches), most volcanoes have clear precursors before eruption (unrest phase), which can last from days to months. Some volcanoes display decades of heightened unrest, especially in the case of caldera systems such as Long Valley, USA and Campi Flegrei, Italy. In addition, while the climactic phase is commonly reached within a week from the beginning of the eruption, many volcanoes have protracted periods of activity potentially lasting months to decades. Finally, many explosive eruptions that produce abundant supplies of lose, unconsolidated sediment on steep volcanic slopes have protracted periods of post-eruption land change when rainfall and wind remobilise the pyroclastic material for years after the end of some explosive eruptions. Collectively, this means that people near volcanoes could potentially be affected over very long time periods during three distinct phases (unrest, eruption, and post-eruption time periods), each with either primary or secondary hazards or both.
Multi-hazard nature of volcanic eruptions
The United Nations quantified the cost of volcanic eruptions at about USD 153 billion between 1995 and 2015 (https://www.weforum.org/agenda/2016/05/costliest-volcanic-eruption-in-history/), with the 2010 eruption of Eyjafjallajökull in Iceland and the 1985 eruption of Nevado Del Ruiz in Colombia being associated with some of the largest economic impact of about USD 3 and USD 1 billion, respectively. Costs can be direct, when related to damage to infrastructure and homes, or indirect, when related to cascading disruption to various economic sectors including industry and transport. Nonetheless, it is important not to 'stereotype disasters' as recommended by PAHO (1999). In fact, not only the impact differs according to the economic, social and political situation in the affected country, and degree to which its infrastructure is developed, but also to the source of disaster.
Unlike other natural phenomena, which may have one or a few associated primary hazardous processes associated with it (e.g., earthquakes, floods), volcanic eruptions are intrinsically almost always associated with multiple hazards. Eruptions can generate a variety of primary hazards including tephra fallout, lava flows, pyroclastic density currents (PDCs), and volcanic gases, as well as secondary hazards such as remobilisation of pyroclastic material by water (which produce lahars) and by wind (which produce ash storms), glacial outburst floods generated by volcanic subglacial eruptions (especially common in Iceland where they are called jökulhlaups) and tsunami created by a range of conditions such as sector collapses and landslides to PDCs entering the ocean and rapidly displacing water (e.g. Bonadonna et al. 2021) (Fig. 1: volcanic hazards; source: USGS). All these different phenomena act at different temporal and spatial scales and are associated with different agents of damage such as temperature, dynamic pressure, static load, toxicity, and chemical attack (e.g. Blong 2000).
Variability of impacts associated with volcanic eruptions
The impacts that each of these volcanic hazards can produce also depend on the vulnerability of the specific exposed elements. This includes human populations, buildings, infrastructures, and natural eco-systems. As an example, the main damage agents of PDCs and lava flows are dynamic pressure and temperature, while the main damage agents of volcanic ash are static load, chemistry and electrical conductivity. Nonetheless, PDCs are significantly faster than lava flows and, therefore, they represent one of the deadliest volcanic phenomena with a high destruction potential (e.g. Cole et al. 2015). Depending on eruption intensity (i.e., volumetric or mass eruption rate), both PDCs and lava flows tend to impact proximal to medial areas up to tens of kilometres from the volcano.
In contrast, volcanic ash has the potential to impact communities up to a few hundred to a few thousand kilometres from the vent, with impacts that range from structural damage of buildings and infrastructures to damage to vegetation and mild to severe health effects in humans and other animals (e.g., Jenkins et al. 2015; https://www.ivhhn.org/information#ash). Volcanic ash is also the main hazard affecting global air traffic as it can cause aircraft engines to lose thrust, stall or completely lose power. The impact of primary dispersal and deposition of volcanic ash can be exacerbated by remobilisation by wind that can last for decades after the end of an eruption and affect significantly larger areas (e.g., Dominguez et al. 2020).
Pyroclastic deposits can also be remobilised by water generating debris flows (also called lahars in volcanology) with the potential to cause significant disruption up to many tens of kilometres from the volcano with the main damage agent being dynamic pressure (e.g., Pierson et al. 2014). Finally, volcanic gases are mostly associated with toxic action on the biological tissues, reduction or displacement of the normal oxygen concentration in breathing air (for asphyxiant gases) and chemical attack (on buildings, vegetation, etc.) (https://www.ivhhn.org/information#gas). It is important to recognise that most of the gas-related deaths have occurred during non-eruptive periods as some volcanoes are associated with long-term degassing. In addition, sulphur gases can also cause the reduction of tropospheric temperature when injected into the stratosphere with a significant impact on the climate (e.g. Langman 2014, Rampino and Self 1984).
Even though volcanic eruptions are intrinsically multi-hazards, the associated hazards mostly depend on the eruptive style, and, more in detail, on the magma rheology. Silica-poor magmas are mostly associated with effusive eruptions and, therefore, with the generation of lava flows and degassing. In contrast, silica-rich magmas are mostly associated with the development of explosive volcanism and corresponding volcanic plumes that inject large volumes of volcanic ash into the atmosphere. These plumes and other features near the vent may collapse under gravitational forces and generate PDCs. Important exceptions exist. As an example, in specific conditions, silica-poor magma can also erupt explosively, and silica-rich magmas can generate lava flows and shorter coulees. Silica-rich magmas can also form lava domes that can eventually collapse due to gravity, forming destructive and deadly PDCs.
Volcanic eruptions can, therefore, be very complex and evolve with time. Some can last a few hours, while others can last decades, although most eruptions last less than six months. This is why a detailed knowledge of volcanic systems that can potentially impact exposed populations and assets is required to develop dedicated and effective risk reduction strategies (e.g. Bonadonna et al. 2018, 2021).
Variability of humanitarian crises associated with different eruptive styles
In order to illustrate the variability of humanitarian crises associated with volcanic eruptions due to the different associated hazards (e.g. volcanic ash, PDCs, lahars, lava flows), a few case studies are reported here. In particular, the difference between explosive eruptions (mostly associated with volcanic ash and/or PDCs and/or lahars) and effusive eruptions (mostly associated with lava flows and gas) is discussed.
Explosive eruptions
The two deadliest volcanic hazards (PDCs and lahars) and with the potentially widest and most variable impact with distance from the volcano (volcanic ash) are typically associated with explosive eruptions. As mentioned earlier, these three phenomena are characterised by different damage agents and have different spatial and temporal distributions. As a result they require very different strategies in relation to both prevention (e.g. hazard assessment, early warning, mitigation measures) and response (e.g. emergency measures, search and rescue).
The volcanic disaster that most impacted DRR strategies worldwide is that associated with the 1985 eruption of Nevado del Ruiz (Colombia). Even the Sendai Framework for Disaster Risk Reduction adopted at the Third UN World Conference on Disaster Risk Reduction in 2015, which emphasises the importance of a paradigm shift from disaster management to disaster risk management, is built on lessons learned from the Nevado del Ruiz disaster. These include the fact that governments need to take responsibility for early warnings and disaster risk management in order to reduce mortality, the number of people affected and economic losses from disasters (https://www.undrr.org/news/volcano-changed-course-disaster-risk-management). In fact, causes of that disaster included the limitations of scientific prediction/detection, the under-advised and inadequately prepared local authorities, the unprepared population, the refusal to accept a possible false alarm, and the lack of will to act on the uncertain information available (Voight et al. 2013). This disaster also prompted the U.S. Agency for International Development (USAID) and the U.S. Geological Survey to create a partnership known as the Volcano Disaster Assistance Program, or VDAP, to respond to international volcanic events (Disaster Risk Reduction: Pounds of Prevention - Colombia - Colombia | ReliefWeb).
In addition, the 1985 Nevado del Ruiz disaster demonstrated for the first time the deadly potential of lahars and the large distances that they can potentially reach. In fact, a relatively small explosive eruption caused pyroclastic material to mechanically mix with the overlying glacier, generating a series of lahars that travelled at high speed through steep and relatively narrow river canyons, reaching villages up to 100km from the volcano in a few hours and killing about 25,000 people. The immediate response activities were similar to those of many other large disasters and included search and rescue; assessment of the damage; emergency medical care for the injured; provision of short-term food and shelter for those displaced; recovery, identification, and burial of bodies; and reestablishment of lifelines. After the immediate post-impact phase, recovery and reconstruction activities mostly included provision of temporary housing and relocation of survivors and local functions away from the most hit area (i.e. Armero town) (National Research Council et al. 1991).
Similar to the Nevado del Ruiz disaster, more recent humanitarian volcanic crises associated with either lahars or PDCs have mostly involved rescue and relief activities as well as provision of temporary housing and relocation of survivors even if with some different dynamics and logistic challenges (e.g. 2005 lahars at Tolimán volcano, Guatemala; 2018 Fuego eruption, Guatemala). In the first case, Hurricane Stan triggered two deadly lahars on the flanks of Tolimán (a dormant volcano) that rapidly reached the alluvial plain, killing more than 1,000 people in the Mayan Indian village of Panabaj on the edge of Lake Atitlán. The area was declared a “mass grave” by the Guatemala authorities (Guatemala survivors homeless year after mudslide - Guatemala | ReliefWeb). The lahars and the intense rains also destroyed most houses and crop lands that represented people’s main source of income. In Tz’utujil, the word Panabaj means “mud and stone”, in reference to the lahars that have occurred in the area throughout history. Traditionally, no one lived in the area affected by the 2005 lahar. People had only moved on to this marginal land due to extreme economic and political pressures, particularly during the 1960-1996 Guatemalan Civil War.
The June 2018 eruption of the extremely active Fuego volcano (located about 40km from Guatemala City) affected more than 1.7 million people with a death toll of about 200 people and 300 people missing. Fatalities were mostly in relation to PDCs that were generated over a few days and travelled up to about 10km from the volcano. More than 12,000 people were evacuated after the explosion with over 4,000 living in emergency shelters. The search and rescue activities were disrupted by the continuous generation of PDCs and lahars due to heavy rain. About 8,500 hectares of corn, bean, and coffee crops were destroyed and ashfall forced the shutdown of the country's primary airport for a few days (https://www.humanitarianresponse.info/fr/operations/latin-america-and-caribbean/document/guatemala-acaps-fuego-volcano-eruption-report).
The humanitarian crisis associated with the 2021 eruption of Soufrière volcano (St Vincent and the Grenadines) was mostly related to response to ash dispersal and fallout even though also potentially deadly pyroclastic density currents and lahars were generated (https://www.ivhhn.org/crisis-management). Early warning systems enabled the timely evacuation of about 23,000 people with no volcano-related deaths reported. For about two weeks, a series of explosions spread volcanic ash across St. Vincent and the Grenadines as well as neighbouring islands (e.g. Barbados, Grenada, St Lucia), disrupting livelihoods and potable water supply and generating public health risks over hundreds of kilometres. Lahars associated with heavy rain were reported for weeks after the beginning of the eruption.
Immediate, priority needs among displaced households included food, health, and water, sanitation, and hygiene assistance, including measures to avoid the spreading of COVID-19. The water system was shut down, so any drinking water had to come from outside the country. The volcanic activity has damaged 80-100% of agricultural lands and forests in the orange and red hazard zones, affecting the country’s main supply of fresh produce (2021_05_14 USAID-BHA Saint Vincent and the Grenadines Volcanic Eruptions Fact Sheet #1.pdf (reliefweb.int)). An important aspect of recovery programs of humanitarian crises associated with the accumulation of large volumes of volcanic ash is clean-up operations and ash disposal, which can be associated with large costs and logistic challenges (e.g., https://www.ivhhn.org/uploads/Hayes_Wilson_Clean-up%20after%20volcanic%20eruptions.pdf; Hayes et al. 2015). The true economic toll of La Soufrière eruption remains unclear and the humanitarian crisis is probably going to last many more months after the end of the eruption. First estimates of local authorities indicate that the eruption caused USD 150 million in infrastructure damage and USD 150 million in agriculture and housing losses. In addition, it will require USD 20-30 million to clean up the islands and about USD 15 million per month to feed and house evacuees (more than 6,000 are registered in emergency shelters).
Effusive eruptions
Prevention as well as response and recovery activities associated with effusive eruptions can be very different with respect to explosive eruptions. In fact, effusive eruptions are typically not associated with high death tolls, but they can, nonetheless, be very challenging as they can last a long time, require the displacement of a large number of people and cause widespread disruption. Main hazards associated with effusive activity are lava flows and volcanic gases. As an example, the 2018 eruption of Kilauea (Hawaii) lasted 107 days, caused an economic cost of about USD 800 million, and the associated lava flows covered about 35 km2 of land destroying about 700 houses (Williams et al. 2020). The geothermal plant which provided one-quarter of the island's electricity, was forced to shut down and was later damaged by lava flows. Some 2,000 people were evacuated but only a few tens of people got injured, including health effects due to exposure to sulphur dioxide gas. Recovery programmes mostly involved resettling and reestablishment of lifelines, although a 'buy-out' of lands directly affected by the lava flows is under way.
Another interesting example of complex volcanic crisis associated with an effusive eruption is that of Nyiragongo volcano in 2002 that exacerbated the ongoing regional ethnic and military conflict in the Democratic Republic of Congo (Komorowski, and Karume 2015). Lava flows moved rapidly down the slope (>10 m/s versus the typical 0.1-3 m/s in Hawaii) and in a few hours destroyed about 13% of the city of Goma (located 17 km away), 21% of the electricity network, 80% of the economic assets, 1/3 of the international airport runway and the housing of 120,000 people. About 500,000 were forced to flee. More than 400 injuries and about 150 deaths were mostly associated with CO2 asphyxiation and with the explosion of a petrol station near the active hot lava flow.
The lesson learnt from the 1977 eruption of the same volcano that had killed about 600 people in 30 minutes (even though the lava flows did not reach Goma), in combination with the presence of a large humanitarian community in Goma, helped to limit the number of fatalities. First, even though key equipment had been vandalised during the years of military conflict, the scientists of the Goma Volcano Observatory successfully interpreted the increasing fumarolic activity and seismic data from distal stations that helped the management of both the unrest phase and the eruption itself. Second, memories of the 1977 eruptions triggered life-saving actions by villagers, including self-evacuation (Human health and vulnerability in the Nyiragongo volcano crisis DR Congo Jun 2002 - Democratic Republic of the Congo | ReliefWeb).
One of the first tasks of the post-crisis response to the 2002 eruption was to establish a modern operational volcano monitoring network and team in order to improve the technical and analytical capabilities of the Goma Volcano Observatory. In fact, a new large lava lake had formed within the 1000m deep crater soon after the eruption that was associated with a significant sulfur, chlorine, and fluorine-rich gas plume (Komorowski, and Karume 2015). A new eruption occurred on 22 May 2021 with lava flows reaching the northern outskirts of Goma causing 32 fatalities. On 27 May 2021, authorities announced the forced evacuation of around 400,000 people from Goma due to the risk of further activity suggested by seismic and ground deformation data (DR Congo: Volcanic eruption in Goma - Situation Report No. 6, as of 30 May 2021 - Democratic Republic of the Congo | ReliefWeb). Lack of clean water, food and medical supplies, as well as electricity have created catastrophic conditions in many places. Health authorities are also worried about outbreaks of cholera with at least 12 suspected cases identified so far (DR Congo: Volcano Nyiragongo - May 2021 | ReliefWeb).
Reduction of volcanic impact
What is described above highlights the importance of the implementation of DRR strategies and of their adaptation to the different volcanic hazards and eruptive styles in order to reduce the impact and mortality associated with volcanic eruptions. Volcanic eruptions have an advantage with respect to other natural phenomena as they are often associated with precursory signals that accompany the rise of magma to the surface (e.g. volcanic earthquakes, temperature and chemical changes of volcanic gas, deformation). These precursors are key to timely evacuations (e.g., Gregg et al. 2015). Nonetheless, these precursors have to be accurately detected, interpreted and communicated to decision makers in order to avoid human losses. As a result, dedicated early warning systems need to be developed next to potentially impactful volcanoes.
Given the diversity of hazard associated with volcanic eruptions, not only the humanitarian response has to be different but also the mitigation and preparedness strategies should be adapted with respect to the different volcanic systems as well as to the different socio-economic conditions, political situation, level of infrastructure and built environments (e.g. Bonadonna et al. 2018, 2021). Mitigation strategies include geological studies, compilation of hazard and risk maps, hazard-based land-use planning, incentive measures (e.g. insurance, catastrophe bond), engineering structures to reduce hazard impact (e.g. volcano shelters, sabo dams), and education of governments, businesses, and the public on measures they can take to reduce loss and injury.
Preparedness strategies include emergency plans, awareness campaigns, early warning (e.g. monitoring, communication to public and authorities), and evacuation drills. It is important to consider that, in order to be inclusive, to build trust, to favour participation, and to answer specific needs, risk assessments and related science-based services and products should be co-designed and co-produced by a variety of stakeholders including not only scientists, but also broader representatives of the local population. They should also be updated regularly in relation to the different phases of the disaster risk cycle. Finally, DRR for volcanic eruptions might also require collaborations across nations as volcanoes produce trans-boundary hazards and risks.
---
Costanza Bonadonna, Department of Earth Sciences, University of Geneva
Costanza.Bonadonna@unige.ch